Because sometimes it’s easier to control a computer than rotation, salinity, water and dye.
After looking at a non-rotating cylinder collapse the other day, it is time to look at proper hetonic explosions (you know? The experiment on the rotating tank where a denser column of water at the center of the tank is released when the whole tank has reached solid body rotation). In Bergen, we used to show this experiment as a “collapsing column” experiment, the tilting of a frontal surface under rotation. For those cases, all the parameters of the experiment, e.g. the rotation rate, the density contrast, the water height, the width of the cylinder, were set up such as to ensure that one single column would persist in the middle of the tank. At JuniorAkademie, we’ve also run it in other setups, to form dipoles or quadrupoles. For a real hetonic explosion, we would typically go for even more eddies than that.
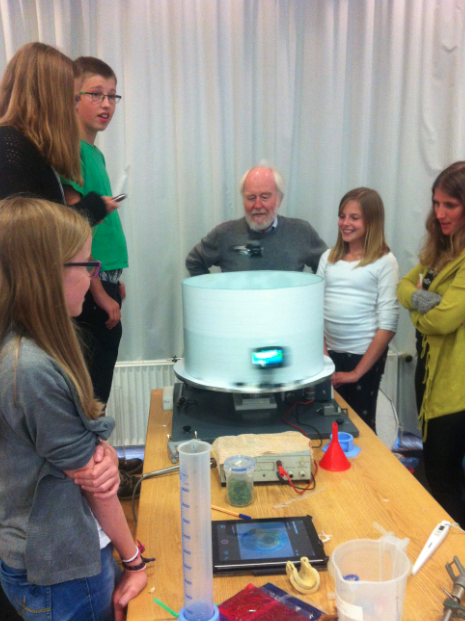
Students watching the experiment shown below. We put paper on the outside of the tank because all the feet swiping past are kind of distracting on the movie later, but that is obviously really annoying for live observers. But in our defense – we only did this once for one experiment late one evening, and didn’t expect so many people to be interested in the experiment! Plus they got to watch on the tablet which showed the top-camera’s view via WiFi… ;-)
But if you read through all those posts then, you might remember that I’ve been complaining about how it is really difficult to set up an experiment in such a way that you have total control over the amount of vortices that form. Firstly, because the system is inherently chaotic, but let’s forget about that for a minute. But then because the calculations aren’t that easy for school kids to do, and then even when everything is calculated correctly, water has to be prepared with the correct salinity, the rotation has to be set to the correct period, the cylinder has to be completely centered in the tank, the water level has to be just right and when the cylinder is pulled up, this has to happen with a swift movement as to add as little disturbance as possible. Not an easy task, especially when there is a camera mounted on the tank!
To show us what to expect, Rolf did some model simulations for us. This is what a monopole looks like:
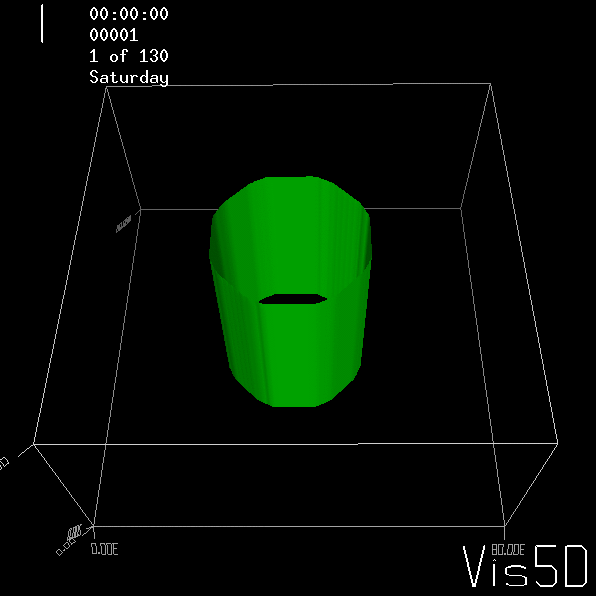
Shown is an isoline in density, separating the dense water below from the lighter water above. Superimposed are the horizontal velocities, so you get a sense of the rotation.
For more advanced experimentalists to recreate, here a dipole:
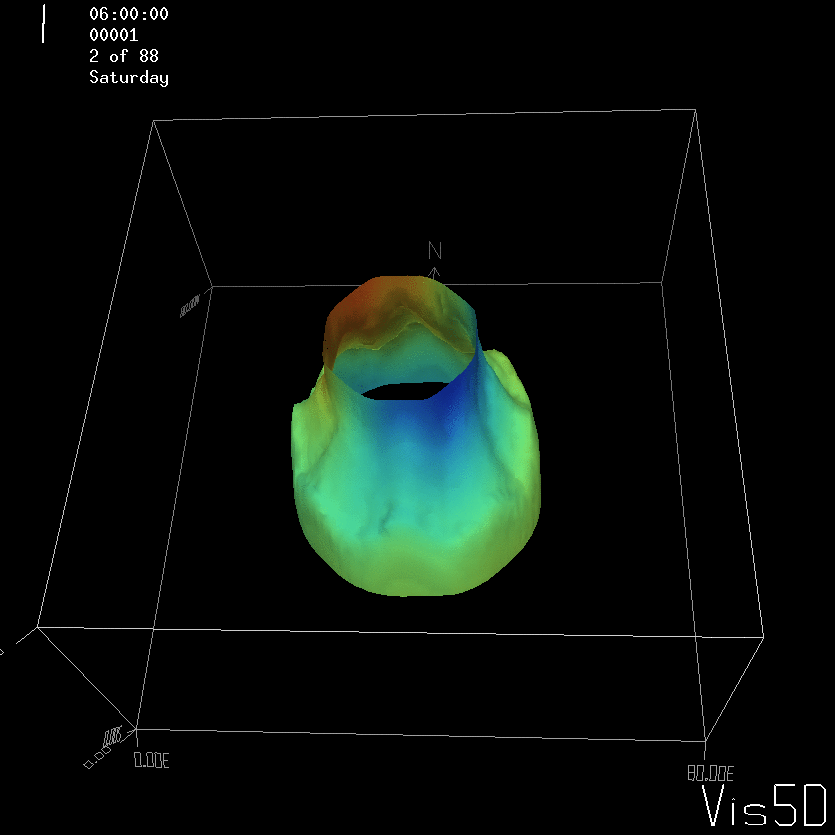
As for the monopole, you see chimneys that are open on top. That is because the density is higher than the one of the isoline inside the eddy, so you get the impression that you can look inside.
But the picture is different for quadrupoles, here the four eddies (that form when the central column breaks up) do not reach the water surface any more, hence they appear closed in the visualization below.
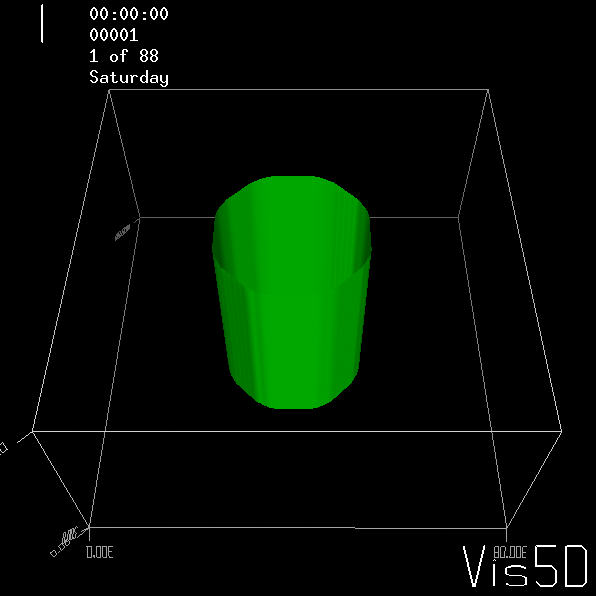
Btw, the time is of course not measured in weekdays, that’s just a glitch in the visualization that we didn’t fix.
Seeing the simulated situations for the three cases above was quite comforting after having run this experiment a couple of times. When you run the experiment in a tank, there is always a lot of turbulence that you wish wasn’t there. But it really helps to keep your expectations in check when you see that in the simulation there are always little vortices, trying to break away from the main ones, too, and that that is how it is supposed to be.
So now for an attempted experimental monopole, which turned out as a dipole due to turbulence introduced when removing the cylinder, similarly to what happened to us in the no-rotation collapsing column experiment.
When you watch the side views closely, you can see that the tank appears to be wobbling (which we usually can’t see, because this is the only time we taped a camera to the side of a tank – usually when filming from the side, I film from outside the rotating system, holding the camera in my hand). You see it most clearly when the yellow dye crystals are added – the water is sloshing back and forth, and that is most definitely not how it is supposed to be. Oh, the joys of experimentation! But what is pretty awesome to see there is how the vertical dye streaks get pulled apart into sheets as they get sucked into the vortices. Reminds me of Northern Lights! :-)