Different types of experiments, and why we use such a weirdly-shaped “Antarctica” and are happy with it.
When we want to show people images of our model experiments in a tank, people often imagine that they will be shown cute little miniature landscapes, looking much like the ones you see for really fancy model train setups. And then they are hugely disappointed when they see pictures like the one below and we tell them that yes! that’s our Antarctica that Nadine is climbing on, while Elin is sitting in the Southern Ocean.
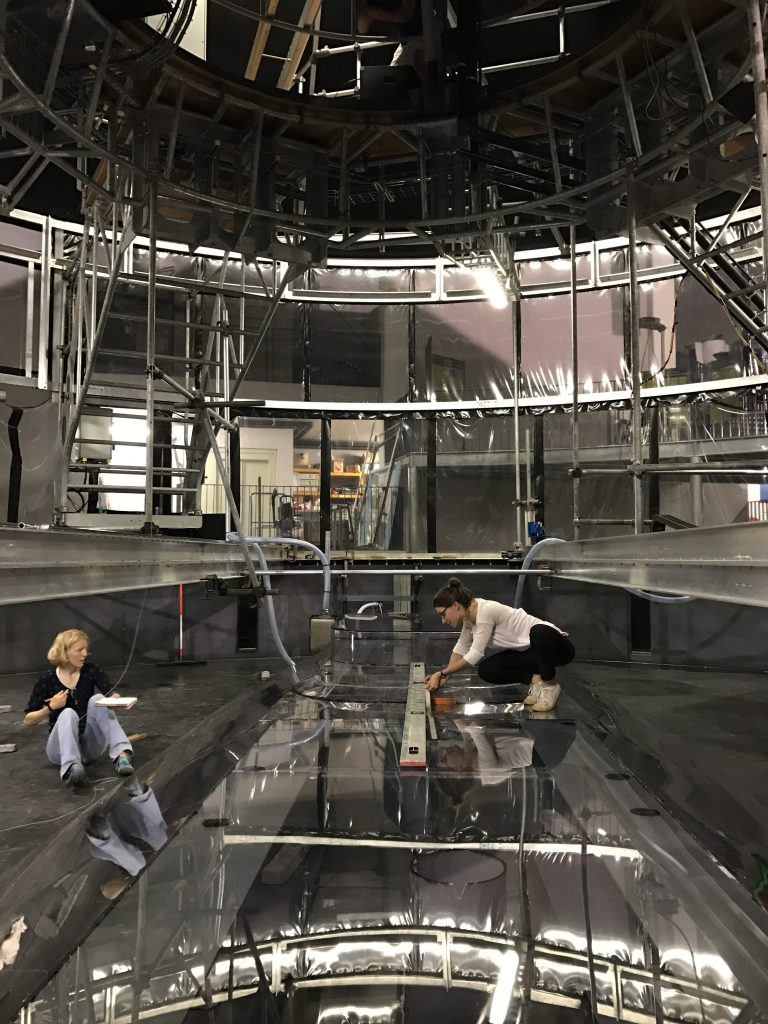
The kind of experiment everybody hopes to see could, according to Faller (1981), be classified as a simulation: representing the natural world in miniature, including every detail. Data from those experiments — since they would in theory be realistic representations of the real world — could be used to fill in missing data from the real world in regions that are hard to get real data from, like for example the Southern Ocean. However, since those experiments are designed to represent the complexity of the real world, interpretation of the experiments is as complex as it is to interpret data from the real world: There are so many processes involved that it is hard to isolate effects of individual processes.
The kind of experiments we are doing would be classified as abstractions. Faller describes this kind of experiment as similar to abstract art: Only the main features, or better: the artist’s interpretation of the main features, are reproduced and everything else is omitted. That makes the art difficult to understand for anyone who isn’t well versed in abstract art, but for the experts it is obvious what the point is.
In case of our experiments that means that we have all the relevant features, or better: our interpretation of what we believe to be relevant features, of Antarctica present in the tank: the parts of topography that we think have an influence on how the current should behave, i.e. a V-shaped canyon, a source that supplies water of the correct properties into the ambient “ocean” water, an ice shelf. And when that ice shelf is tilted, we feel like our experiments are already becoming pretty realistic!
These abstractions are the kinds of experiments in which you can, because they are relatively simple, develop new theories when new features of the circulation emerge that you then have to rationalize and include in your theories after the fact.
We have actually also done another type of experiment, a verification. I wrote about it in this post: we tilted the ice shelf because this is a case for which we actually knew from theory how our current should behave, in contrast to all the previous experiments where we didn’t actually know what to expect, and we were happy when we observed exactly what we expected based on theoretical considerations. So in this case the experiment wasn’t about discovering something new, but rather making sure that our understanding of theory and what goes on in the tank actually match.
Faller describes a last type of experiment: the extension. That is the kind of experiment that you could perform after a successful verification experiment: Pushing the boundaries of the theory. Does it still hold if the current introduced in the tank is very fast or very slow? If the water is very deep? If the slope of the ice shelf is very large or small? Basically, every parameter could now be changed until we know for which cases the theory holds, and for which it does not.
So why am I writing all of this today? Faller’s (1981) article, before he goes on to describe the framework to think about geophysical fluid dynamics experiments that I mentioned above and which I find quite helpful to consider, starts with the sentence “No one believes a theory, except the theorist. Everyone believes an experiment — except the experimenter.” On this blog, our goal is to bring the two together and not make anyone believe either of them, but to show how both can work together to mutual benefit.
Faller, A. J. (1981). The origin and development of laboratory models and analogues of the ocean circulation. Evolution of Physical Oceanography, 462-479.