Hydraulic jumps, especially submerged ones, are a very theoretical concept for many students, one that occurs in a lab experiment if they are lucky, but more likely only seems to exists in videos, drawings, and text books. But we can observe them all the time if we know what we are looking for! They don’t only occur in hard-to-see places like the Denmark Strait (for you oceanographers) or inside some big plant, mixing in one chemical or another (for you engineers), they are everywhere!
So. Submerged hydraulic jumps. You don’t think about them for years and years, then one day a friend (Hi, Sindre!) asks about them and the next day you come across this:
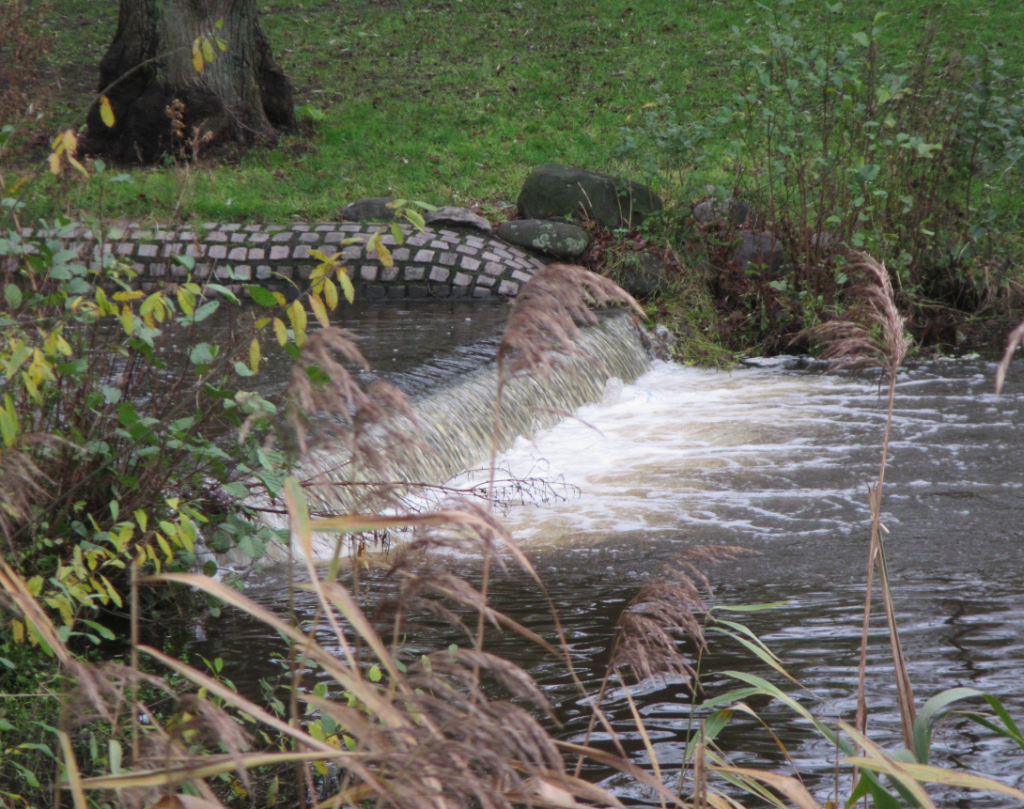
A tiny waterfall in Schleswig
A tiny waterfall that not only shows a beautiful submerged hydraulic jump, but provides extra entertainment in the form of two empty bottles caught up in the return flow above the submerged hydraulic jump:
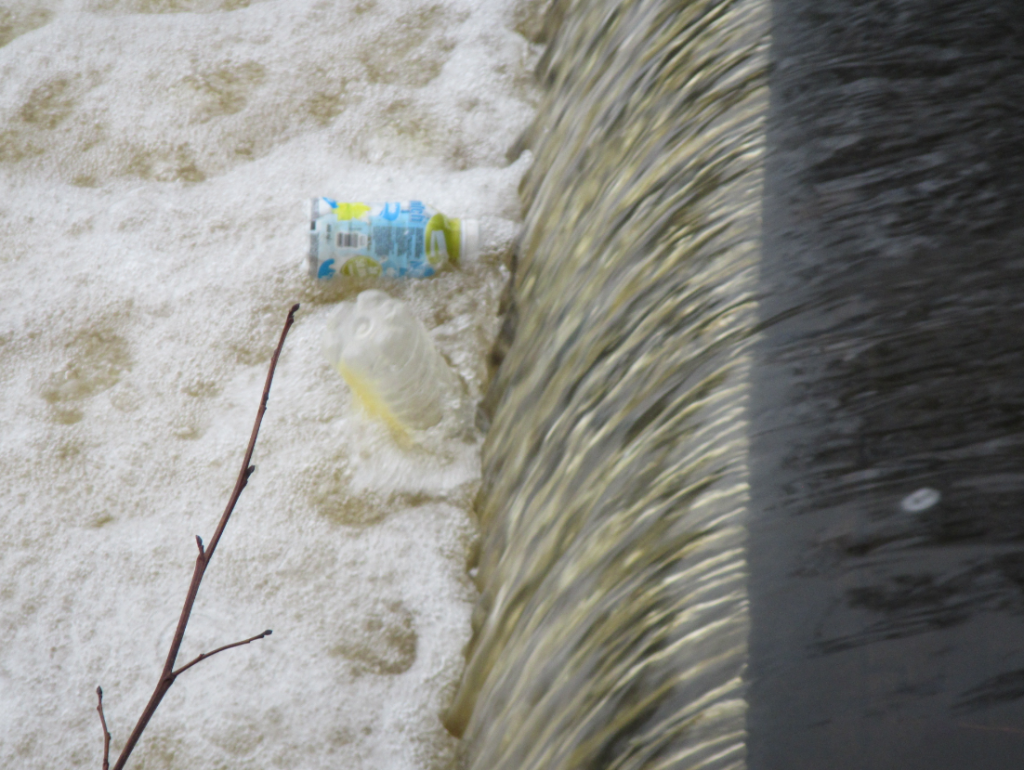
Litter caught up in the return flow above a submerged hydraulic jump
You should watch the video, it is really entertaining!
So what is going on here? Below a sketch: Water from the reservoir (A) flows down over a sill. It actually doesn’t flow, but it shoots (B), meaning that it flows faster than waves can propagate. Any wave in the flow that would normally propagate in all directions now cannot propagate upstream any more and is just flushed downstream. At (C), the flow has slowed down enough again that wave speed is the same as flow speed, we are at the hydraulic jump. In this case it is submerged – meaning that it occurs below the water’s surface. We can also think of non-submerged hydraulic jumps – see for example here. But what also happens with submerged hydraulic jumps is that the water jet shooting down the slope is so fast that it entrains water from outside the jet and pulls it down with it. This water has to come from somewhere, so we get a return flow (D). And this is exactly where the bottles are caught: In the flow that goes back towards the jet shooting down the slope.
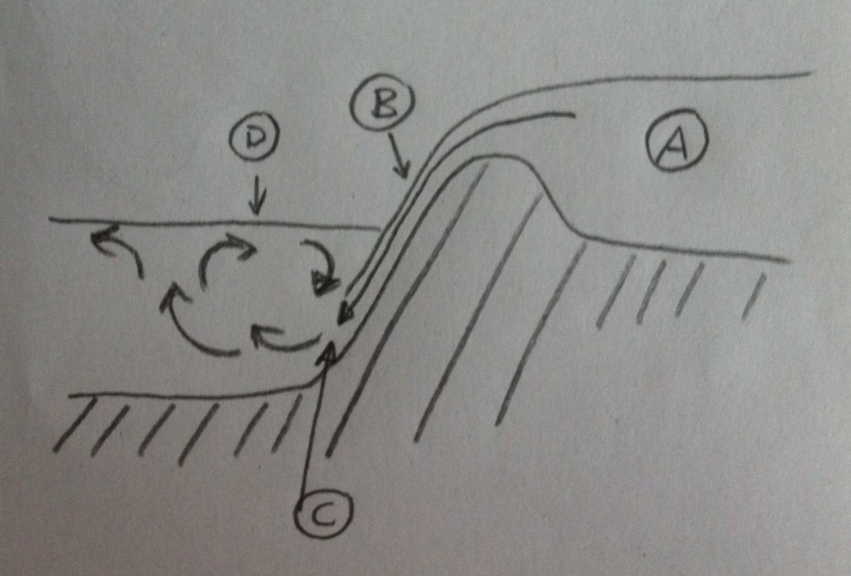
Sketch of the submerged hydraulic jump. A: reservoir. B: water shooting down the slope. C: hydraulic jump. D: turbulent return flow.
When the bottles come too close to the jet, they get pulled under water and then “jump” because they are too buoyant to actually sink. They might jump away a little from the jet, but as you see in the movie, the return flow reaches out quite a bit from where the jet enters the water, trapping the bottles.
This is actually what makes man-made waterfalls so dangerous: You saw in the movie that the return flow pattern is very similar over the whole width of the “waterfall”. So anything trapped in there will have a really hard time getting out. If either the sill or the slope were a little more irregular, it might break up the symmetry and allow things (and animals or people) to get out more easily. Of course, in this case the drop isn’t very high, but imagine a larger weir. Not fun to get caught in the return flow there!
Talking to my Norwegian friends about these things and especially using movies from my reality to illustrate concepts always makes me want to apologize for how tiny our waterfalls are, how in the middle of a city everything is, how much litter there is everywhere, how regulated even the tiniest streams around here are. But then I realize that it is actually really cool that even in the middle of the city we can spot all this. You don’t need the wide open, pristine nature to get yourself – and your students! – excited about oceanographic phenomena!