More physics applications connected to tea.
After the frustrations of taking pictures of steam in my last post, I decided that I could use the very same cute mug to show other stuff, too. I know it has been done over and over again, but we have new students every year, don’t we, so someone has to keep telling the old stories, right?
So. When should you pour the milk into your tea? Right away or a little later?
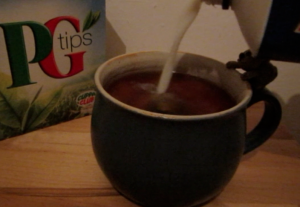
Milk and tea
The answer, as you know, is “it depends”.
Do you want your tea as hot as possible? Then put the milk in right away and it won’t cool the tea down as much. Want the milk to cool down the tea as much as possible? Then wait for as long as you can before pouring it in.
The explanation behind this is of course that the cooling due to evaporation is happening faster the larger the temperature difference between the tea and the surrounding air. If you let it sit without milk, due to the larger temperature difference it cools down faster than if you poured in the cold milk, thus cooling it closer to room temperature, and then waited.
And there are even occasions when you would you put milk into the cup before adding the tea: If you have delicate china and don’t want to risk ruining it by pouring in almost boiling tea. Plus allegedly that way the milk doesn’t scald and form those weird flakes?