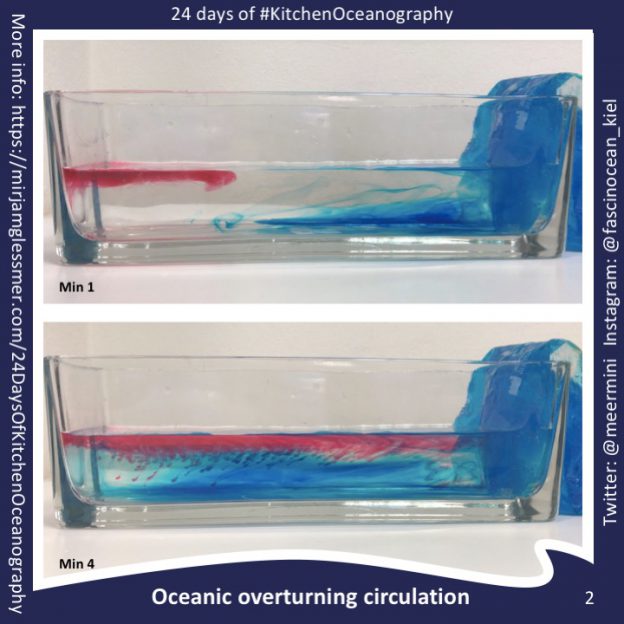
Tag Archives: overturning
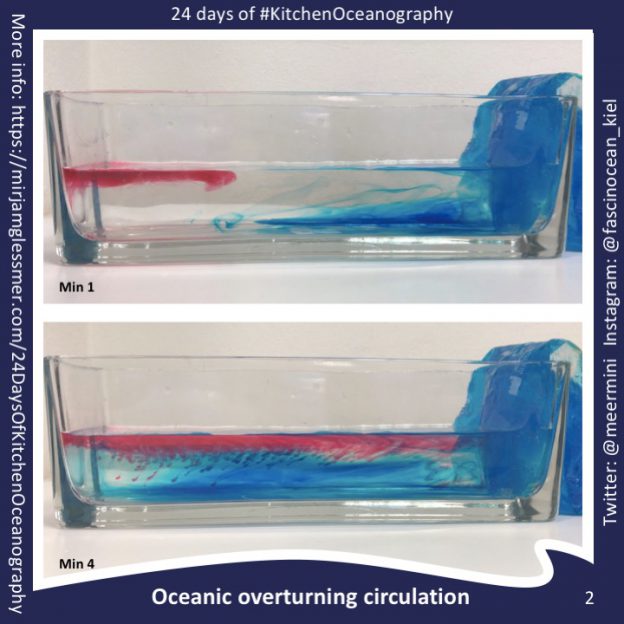
Thermal forcing vs rotation tank experiments in more detail than you ever wanted to know
This is the long version of the two full “low latitude, laminar, tropical Hadley circulation” and “baroclinic instability, eddying, extra-tropical circulation” experiments. A much shorter version (that also includes the end cases “no rotation” and “no thermal forcing”) can be found here.
Several of my friends were planning on teaching with DIYnamics rotating tables right now. Unfortunately, that’s currently impossible. Fortunately, though, I have one at home and enjoy playing with it enough that I’m
- Playing with it
- Making videos of me playing with it
- Putting the videos on the internet
- Going to do video calls with my friends’ classes, so that the students can at least “remote control” the hands-on experiments they were supposed to be doing themselves.
Here is me introducing the setup:
Today, I want to share a video I filmed on thermal forcing vs rotation. To be clear: This is not a polished, stand-alone teaching video. It’s me rambling while playing. It’s supposed to give students an initial idea of an experiment we’ll be doing together during a video call, and that they’ll be discussing in much more depth in class. It’s also meant to prepare them for more “polished” videos, which are sometimes so polished that it’s hard to actually see what’s going on. If everything looks too perfect it almost looks unreal, know what I mean? Anyway, this is as authentic as it gets, me playing in my kitchen. Welcome! :-)
In the video, I am showing the two full experiments: For small rotations we get a low latitude, laminar, tropical Hadley circulation case. Spinning faster, we get a baroclinic instability, eddying, extra-tropical case. And as you’ll see, I didn’t know which circulation I was going to get beforehand, because I didn’t do the maths before running it. I like surprises, and luckily it worked out well!
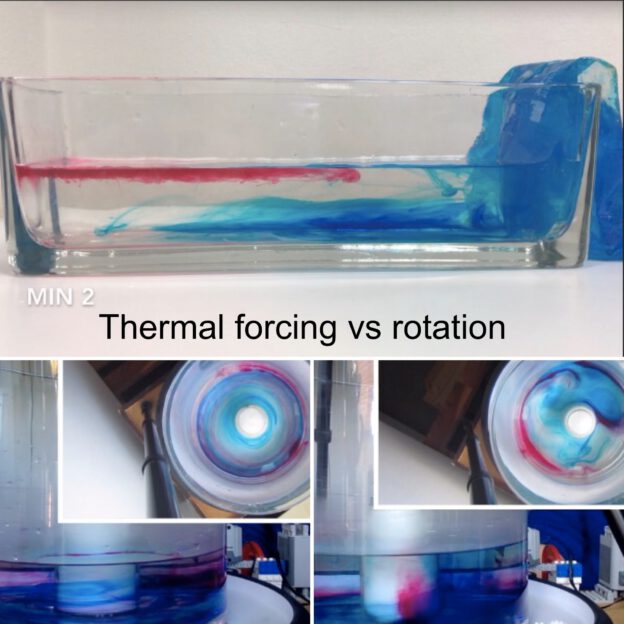
Thermal forcing vs rotation
The first experiment we ever ran with our DIYnamics rotating tank was using a cold beer bottle in the center of a rotating tank full or lukewarm water. This experiment is really interesting because, depending on the rotation of the tank, it will display different regimes. For small rotations we get a low latitude, laminar, tropical Hadley circulation case. Spinning faster, we get a baroclinic instability, eddying, extra-tropical case. Both are really interesting, and in the movie below I am showing four experimentsm ranging from “thermal forcing, no rotation”, over two experiments which include both thermal forcing and rotation at different rates to show both the “Hadley cell” and “baroclinic instability” case, to “no thermal forcing, just rotation”. Enjoy!
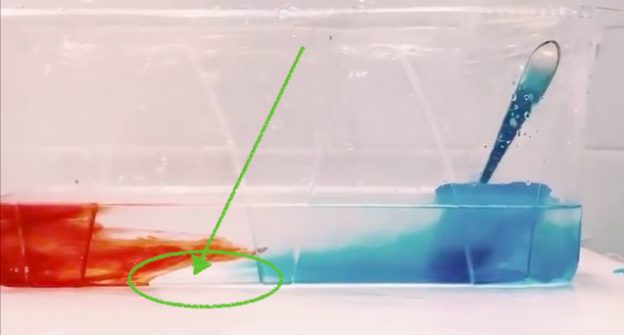
Brine rejection and overturning, but not the way you think! Guest post by Robert Dellinger
It’s #KitchenOceanography season! For example in Prof. Tessa M Hill‘s class at UC Davis. Last week, her student Robert Dellinger posted a video of an overturning circulation on Twitter that got me super excited (not only because as of now, April 15th, it has 70 retweets and 309 likes. That’s orders of magnitude more successful than any kitchen oceanography stuff I have ever posted! Congratulations!).
Robert is using red, warm water on one side and melt water of blue ice cubes on the other side to provide heating and cooling to his tank to create the overturning. Why did I get so excited? Because of this: the head of the meltwater plume was very clearly not blue (see above)! Rob kindly agreed to write a guest post about these observations:
“I first and foremost want to start off by thanking Dr. Mirjam Glessmer for doing a phenomenal job at SciCom through Kitchen Oceanography. I was able to replicate her physical oceanography experiment regarding oceanic overturning circulation for my oceanography class with Dr. Tessa Hill.
As mentioned in her previous post, oceanographic currents are often simplified to give an easier understanding of how oceanic overturning circulation operates. The top 10% of our oceans are controlled by wind-driven currents and tidal fluctuations while the bottom 90% of our ocean currents are controlled by density-dependent movements.Originally this process was defined as thermal circulation but was later expanded to thermohaline circulation. Thermohaline circulation is dependent upon both temperature (thermal) and salinity (haline.) These density-dependent reactions occur when either freshwater fluxes meet saltwater and from thermal differences in water masses. Due to differential heating in our planet, colder formations of dense water masses are formed at the poles, which in turn causes the convective mixing and sinking of water masses driving oceanic circulation.
(Video by Robert Dellinger; thanks for letting me use it!)
In this experiment, we primarily focus on the thermally dependent reactions between two water masses. As seen in the video, the warmer water mass is dyed red, while the cold water mass formed by ice melting, is blue. As expected the more dense water mass (cold) is pushed under the warmer mass once they meet. One feature I would like to point out is the clear plume head feature in my experiment (see picture on top of this post). My theory is that part of the ice cube that was not dyed melted first and was pushed under the warm water mass. This feature is most likely due to the ice cube experiencing unequal cooling, which in turn led to an uneven dye distribution as seen in the previous post “Sea ice formation, brine release, or: What ice cubes can tell you about your freezer.”
As seen in the experiment, thermohaline circulation is thermally driven therefore, the role of salinity causes the system to be non-linear. Salinity serves as a positive feedback mechanism by increasing the salinity of deeper water and strengthens the circulation. Furthermore, current studies are focusing on how atmospheric warming is altering thermohaline circulation attributed by increases in ocean heat absorption and freshwater fluxes (primarily from melting ice caps.)”
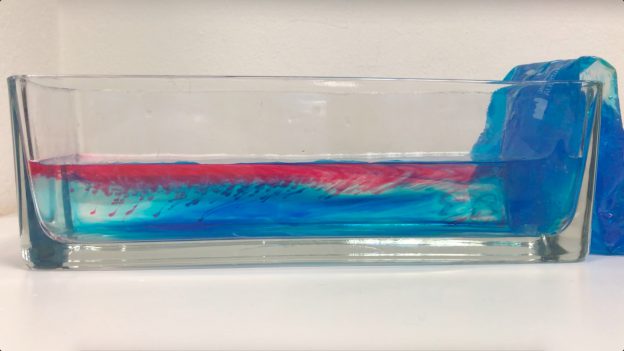
Salt fingers in my overturning experiment
You might have noticed them in yesterday’s thermally driven overturning video: salt fingers!
In the image below you see them developing in the far left: Little red dye plumes moving down into the clear water. But wait, where is the salt? In this case, the “double” in double diffusion comes from heat and dye which are diffusing at different rates. As temperature’s molecular diffusion is about 100x faster than that of salt (or other things that have to physically change their distribution, rather than just bump into each other to transfer energy), the red and clear water quickly have the same temperature, but then the red dye makes the red water more dense, hence it sinks.
Over time, those fingers become more and more clearly visible…
Until after a couple of minutes, we see that they are really contributing to mixing between the two layers.
Even though double diffusive mixing happens in the ocean, too, the scaling of these fingers is of course totally off if we think of this tank as for example the northern half of the Atlantic. But then so is the density stratification… But it’s always good to keep in mind that while this experiment is showing some things quite nicely, there are also things that are artefacts of the way the experiment is set up and that aren’t analogous to how things work in the ocean.
A really nice and very new-to-me way of observing them is from above:
This is a picture that was taken fairly early in the experiment, when the layers hadn’t propagated far yet and the salt fingers weren’t being pulled back by the shear between the layers. But it’s nice to see how the dye is concentrated in those downward moving fingers, isn’t it?
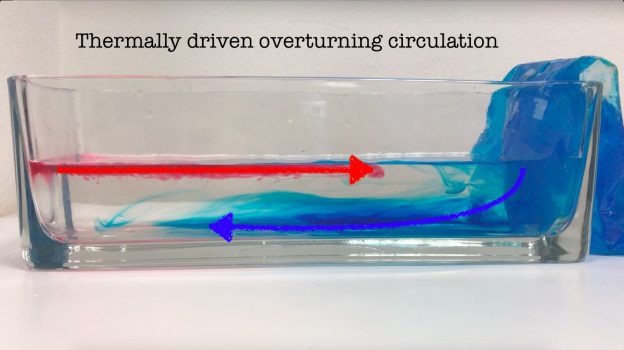
Thermally driven overturning circulation
Today was the second day of tank experiments in Torge’s and my “dry theory 2 juicy reality” teaching innovation project. While that project is mainly about bringing rotating tanks into the theoretical teaching of ocean and atmosphere dynamics, today we started with the non-rotating case of a thermally driven overturning circulation.
Very easy setup: A rectangular glass vase filled with luke-warm water. A frozen cool pack for sports injuries draped over one end (which we’ll think of as the northern end) provides the cooling that we need for deep water formation. The deep water is conveniently dyed blue with food dye. Red food dye is warmed up and added to the “southern end” of the tank, and voilà! An overturning circulation is set up.
Watch the sped-up movie to see what happens:
As you will notice, this circulation won’t last for a very long time. Since we are adding neither warming nor mixing, the cold water will eventually fill up the tank. But it’s still quite a nice experiment!
(And should you have noticed the “salt fingers” forming towards the end of the movie, I’ll write about those tomorrow)
And here is the nice group of students that humoured me and posed for this picture. It’s fun with such a motivated group that comes up with new things to try all the time! :-)
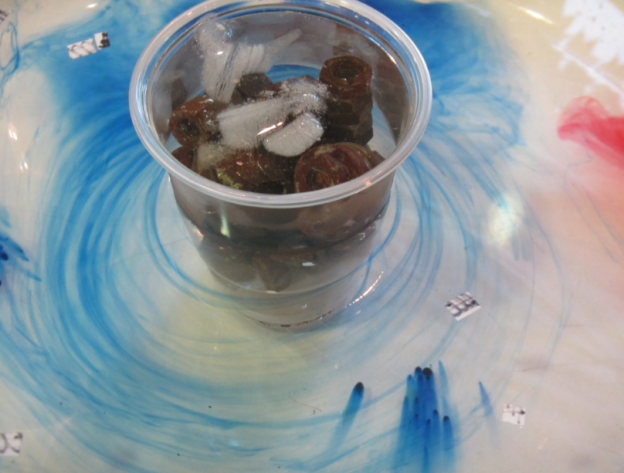
Combining a slowly rotating water tank with a temperature gradient: A thermal wind demonstration!
Setting up an overturning circulation in a tank is easy, and also interpreting the observations is fairly straightforward. Just by introducing cooling on one side of a rectangular tank a circulation is induced (at least for a short while until the tank fills up with a cold pool of water; see left plot of the image below).
But now imagine an axially symmetric setup where the cooling happens in the middle. What will happen to that overturning circulation if the tank is set into rotation (see right plot above)?
First, let’s check there is an overturning circulation. We can see that there is when we look at dye crystals that sank to the bottom of the tank: Dye streaks are moving outwards (and anti-clockwise) from where the crystals dropped on the ground, so at least that part of the overturning circulation is there for sure. If our tank were taken to represent the Hadley cell circulation in the atmosphere, this bottom flow would be the Trade winds.
Now, in addition to having water sink in the middle of the tank, spread radially outwards, and returning by rising near the outer edge of the tank and flowing back towards the middle, a secondary circulation is induced, and that’s the “thermal wind”. The thermal wind, introduced by the temperature gradient from cold water on the inside of the tank to warmer waters towards the rim, tilts columns that would otherwise stay vertically.
You see that in the image below: Dye dropped into the tank does not sink vertically, but gets swirled around the cold center in a helix shape, indicated in the picture below by the white arrows. In that picture, the swirls are tilted very strongly (a lot stronger than we’d ideally have them tilted). The reason for that is that we just couldn’t rotate the tank as slowly as it should have been, and the higher the rotation rate, the larger the tilt. Oh well…
So this is the current pattern that we observe: An overturning circulation (sketched with the red arrows representing warmer water and the blue arrows representing colder waters below), as well as the thermal wind circulation (indicated in green) with stronger currents near the surface (where the water is moving in the same direction as the rotating tank, but even faster!) and then a backward flow near the bottom. The velocities indicated here by the green arrows are what ultimately tilted our dye streaks in the image above.
The thermal wind component arises because as the overturning circulation moves water, that water carries with it its angular momentum, which is conserved. So water being brought from the rim of the tank towards the middle near the surface HAS to move faster than the tank itself the closer it gets to the middle. This flow would be the subtropical jets in the Hadley cell circulation if out tank were to represent the atmosphere.
Here is an old video of the experiment, first shown 5 years ago here. I’m looking forward to when Torge’s & my rotating tanks are ready so we can produce new videos and pictures, and hopefully being able to rotate the tank even more slowly than we do here (but that was the slowest possible rotation with the setup we had at that time). I promise you’ll see them here almost in realtime, so stay tuned! :-)
Experiment: Oceanic overturning circulation (the slightly more complicated version)
The experiment presented on this page is called the “slightly more complicated version” because it builds on the experiment “oceanic overturning circulation (the easiest version)” here.
Background
One of the first concepts people hear about in the context of ocean and climate is the “great conveyor belt”. The great conveyor belt is a very simplified concept of the global ocean circulation, which is depicted as a single current that spans the world oceans (see Figure 1 below). In this simplified view of the global circulation, water flows as a warm, global surface current towards the North Atlantic, where it cools, sinks and finally returns southward and through all the world oceans near the bottom of the ocean. Water is transported back to the surface through mixing processes and starts over its journey again as a warm surface current. While in reality some part of the conveyor belt is wind-driven and many processes come to play together, a large part of the circulation can be explained by the water sinking due to cooling at high latitudes.
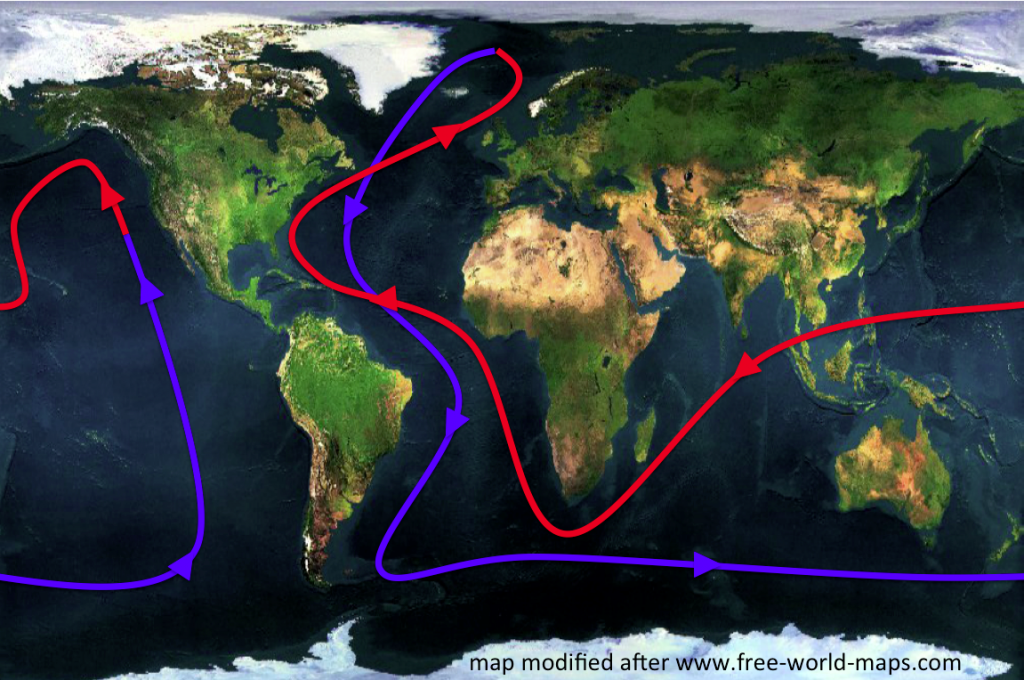
Figure 1: The great conveyor belt. My sketch on top of a map from http://www.free-world-maps.com
This can be very easily represented in a demonstration or experiment.
Materials
What we need for this experiment:
- 2 gel pads for sports injuries, one hot, one cold
- red and blue food coloring
- a clear plastic container to act as tank
- a pipette or drinking straws to disperse drops of dye
- dye crystals to show the circulation. Can also be drops of a different color dye.
Running the experiment
The container is filled with lukewarm water. On the “poleward” end, we add the cold pad, the warm one at the “equatorward” end of the tank.
Blue dye is tripped on the cold pad to mark the cold water, red dye on the warm pad as a tracer for warm water.
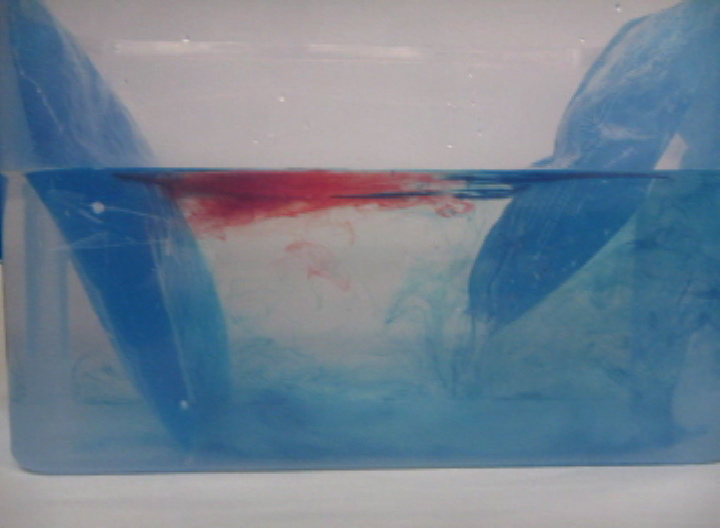
Thermally-driven overturning circulation: Warm water flowing near the surface from the warm pad on the left towards the right, cold flow from the cool pad at the bottom right to left.
A circulation develops. If you drop dye crystals in the tank, the ribbon that formed gets deformed by the currents for yet another visualization of the flow field.
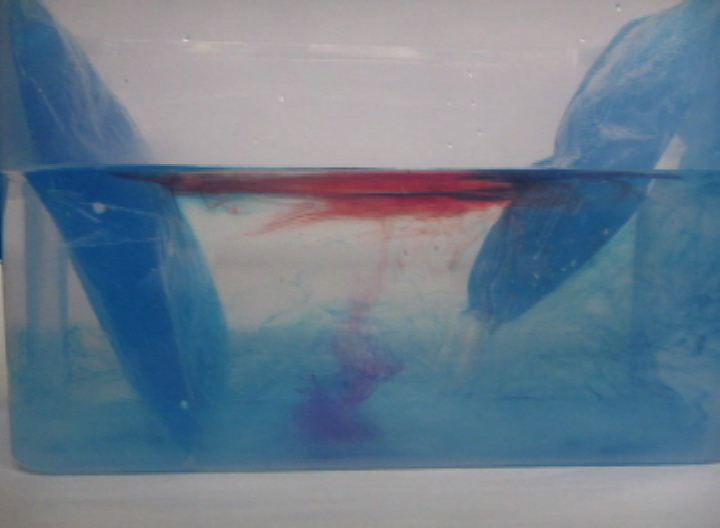
Thermally-driven overturning circulation. In the middle of the tank you see a ribbon of dye, caused by falling dye crystals, being transformed by the currents in the tank.
Here is the video:
What observations to make
Besides the obvious observation, watching, there are a couple of things you can ask your audience to do.
For example, if they carefully slide their fingers up and down the side of the tank, they will feel the warm water near the surface and the cold water at the bottom.
If you have a clear straw, you can use it as plunging syphon to extract a “column” of water from the middle of the tank, showing again the stratification of red, clear, blue.
If you put little paper bits on the surface, you will see them moving with the surface current.
Can you come up with more?
Who can I do this experiment with?
Someone recently asked me whether I had ideas for experiments for her course in ocean sciences for non-majors. Since most of the experiments I’ve been showing on this blog were run in the context of Bachelor or Master oceanography-major courses, she didn’t think that the experiments were as easily transferable to other settings as I had claimed.
So here is proof: You can do pretty complex experiments with non-university level students. To prove my point, let’s go to a primary school.
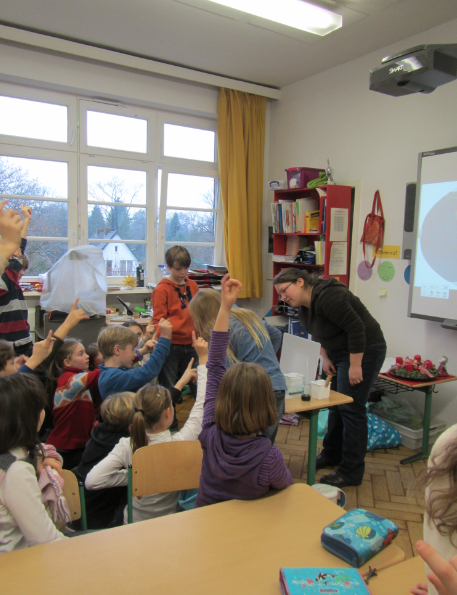
Me running the overturning experiment with a primary school class in 2012.
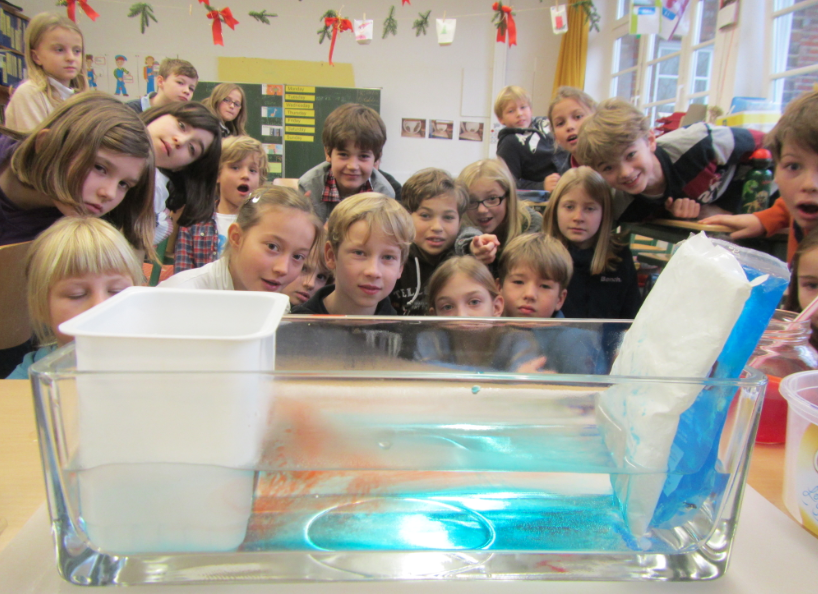
The overturning experiment as seen by the teacher (2012).
Of course, you can adapt this experiment to different levels of prior knowledge. For example, in the primary school, I introduced this experiment by showing pictures of lions and penguins and other animals that the pupils knew live in warm or cold climates, and we talked about where those animals live. In the end this aimed at how temperatures are a lot colder at the poles than at the equator. This is the differential heating we need for this experiment to work. While this is something that I felt the need to talk about with the primary school kids, this can be assumed as a given with older students (or at least that is the assumption that I made).
With the university-level courses, one of the points that I made sure came up during the discussion are the limitations of this model. For example that we apply both heating and cooling over the full depth of the water column. How realistic is that? Or the fact that we heat at one end and cool at the other, rather than cooling on either end and heating in the middle?
Let me zoom in on something in the picture above.
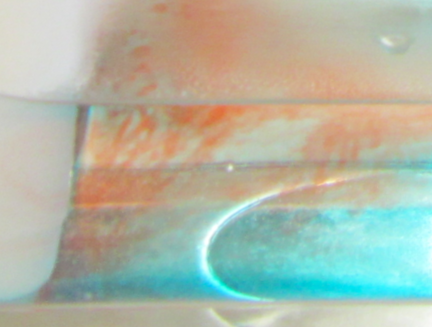
Curious features in the thermal conveyor experiment. Do you know what this is about?
Do you see these weird red filaments? Do you think they are a realistic part of the thermal circulation if it was scaled up to a global scale?
Of course not. What we see here is salt fingering. This is a process that is caused by the different diffusivities of heat and of the red dye. And while it is pretty large scale in our small tank, you cannot scale it up just like that when talking about the real ocean. And it is also really difficult to get rid of salt fingers for this experiment, in fact I haven’t yet managed. But I am open to suggestions! :-)
Another point that I would talk about with university-level students that I would probably not bring up with primary school kids (- although, why not if I had more time than just those 45 minutes per class?) is that ocean circulation is driven by more than just differential heating. Even when just considering the density-driven circulation, that is additionally influenced by changes in salinity. Put that together with wind-driven circulation and we are starting to talk about a whole new level of complicated…
But anyway. My point is that even primary school kids can benefit from doing this kind of experiments, even if what they take away from the experiments is not exactly the same as what older students would take away.
Discussion
—
P.S.: This text originally appeared on my website as a page. Due to upcoming restructuring of this website, I am reposting it as a blog post. This is the original version last modified on January 13th, 2016.
Experiment: Oceanic overturning circulation (the easiest version)
“The easiest” in the title of this page is to show the contrast to a “slightly more complicated” version here.
Background
One of the first concepts people hear about in the context of ocean and climate is the “great conveyor belt”. The great conveyor belt is a very simplified concept of the global ocean circulation, which is depicted as a single current that spans the world oceans (see Figure 1 below). In this simplified view of the global circulation, water flows as a warm, global surface current towards the North Atlantic, where it cools, sinks and finally returns southward and through all the world oceans near the bottom of the ocean. Water is transported back to the surface through mixing processes and starts over its journey again as a warm surface current. While in reality some part of the conveyor belt is wind-driven and many processes come to play together, a large part of the circulation can be explained by the water sinking due to cooling at high latitudes.
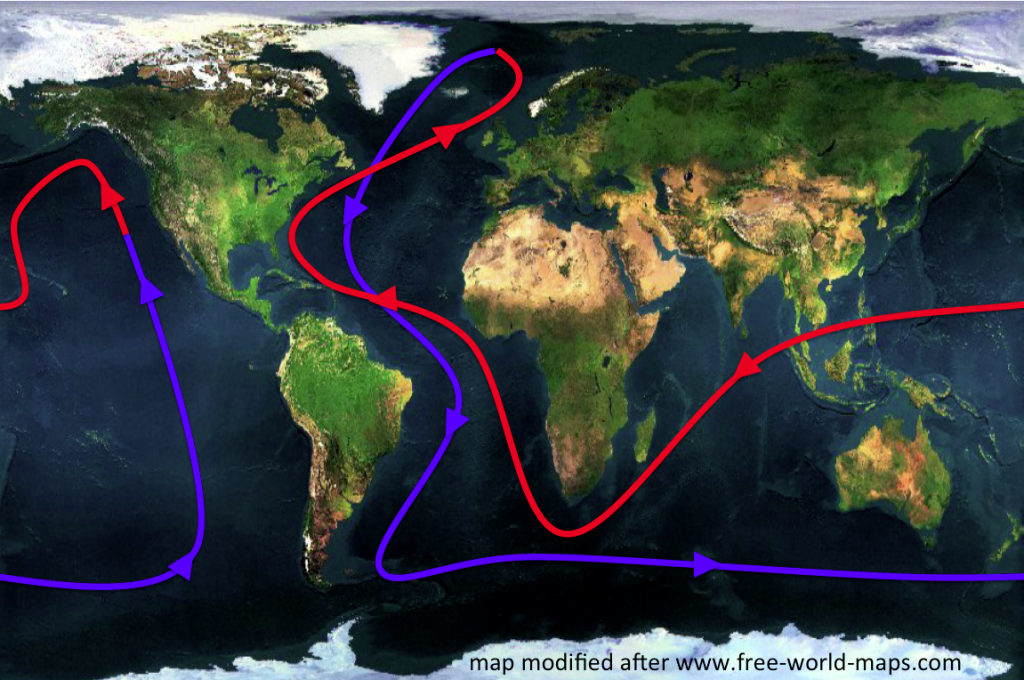
Figure 1: The great conveyor belt. My sketch on top of a map from http://www.free-world-maps.com (used with permission)
The experiment
Since the global conveyor belt is such a basic concept that we come across in many different contexts, it is very useful to have a good demonstration of what is happening in the world ocean. Plus demonstrations and experiments are always fun!
I here present a very simple experiment that can be used for many different purposes. In science outreach, for example on a fair or in a talk, to catch people’s attention and raise an interest in oceanography. In schools to do the same, or to connect the fascination of the ocean to school physics and talk about density, convection, heat. At university to do all of the above, as well as to practice writing lab reports, talk about the scientific method or the validity of simplifications in theoretical or physical models.
Materials needed
All we need to run this experiment is
- a clear plastic container
- lukewarm water
- red and blue food dye
- an ice cube tray and
- access to a freezer.
Ideally we’d also have a thermos or some other kind of insulation to keep the ice cubes frozen until we start running the experiment. To prepare the experiment, all we need to do a half a day ahead is mix some blue food dye into the water that we put in the ice cube tray, and freeze the ice cubes.
Running the experiment
To run the experiment, we start out by filling our “tank” with lukewarm water. Let it settle for a bit. Now we decide for one end of your tank to be the “equator” end. There, we add some red food dye (see Figure 1).
Then we add the blue ice cubes to the “poleward” end of our tank (see Figure 3).
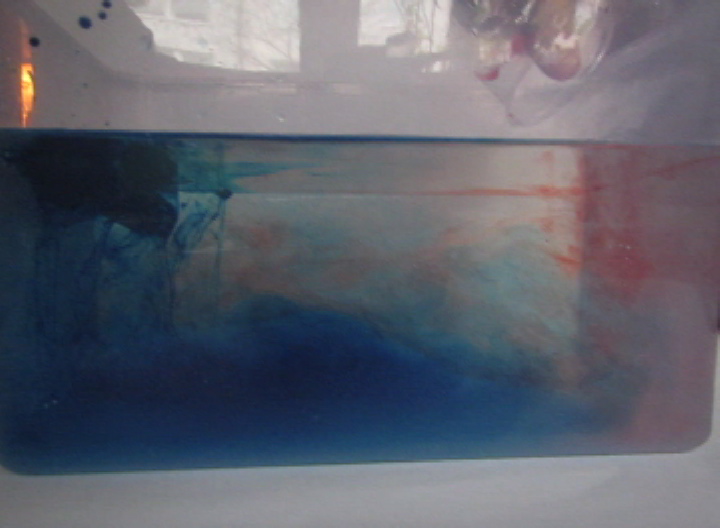
Figure 3: Blue ice cubes melting at the poleward end of the tank. The cold melt water sinks to the bottom of the tank and then spreads “equatorward”.
The cold melt water from the ice cubes is denser than the lukewarm water in the tank and therefore sinks to the bottom of the tank where it spreads “equatorward”, pushing below the warmer water. This can be seen where the red water is pushed upwards and “poleward”.
Discussion
Of course, the processes at play here are not exactly the same as in the real ocean.
For one, deep water formation is NOT due to ice cubes melting in lukewarm water. In fact, melting of sea ice will in most cases not lead to any kind of sinking of water, since the melt water is fresh and the surrounding ocean water is salty and hence denser than the melt water. Cooling in the ocean happens through many processes at the surface of the ocean, like radiation into space and evaporation.
Heating is also represented in an extremely simplified way in this experiment. Heating in the ocean occurs mainly (with the negligible exception of thermal springs in the ocean) by radiative heating from the sun, and at the surface only. We “heat” throughout the whole depth of the ocean by filling the whole tank with lukewarm water.
Also, the mixing processes that, in the real ocean, bring deepwater back to the surface are not represented here at all. Our tank will eventually fill with a layer of cold water at the bottom (See Figure 4), and the circulation will stop once all the ice has melted.
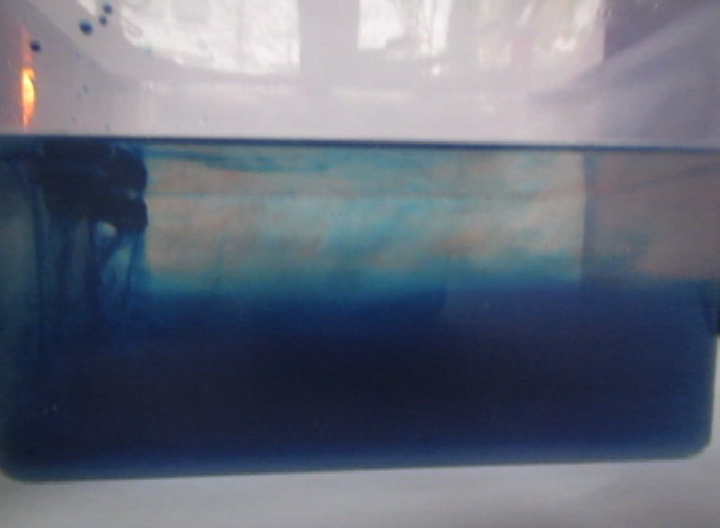
Figure 4: Blue ice cubes melting at the poleward end of the tank. The cold melt water sinks to the bottom of the tank and then spreads “equatorward”. Slowly, the tank fills with cold water.
Why use the experiment?
Even with all the simplifications described above, this experiment is a great first step in becoming intrigued by the ocean, and towards understanding ocean circulation. Seeing the melt water sink from the ice cubes is fascinating no matter how little interest one might have in the physics that cause it. Sliding a finger up and down the side of the tank lets you experience – feel! – how the temperature changes from warm near the surface to very cold near the bottom. Actually physically feeling this is a lot more impressive than just watching the experiment or even just being shown temperature sections of the ocean. And the experiment invites you to play: What if you added little pieces of paper on the water surface, would you see them move with the flow towards the cold end of the tank? Or if you dropped a dye crystal in the middle of the tank, would the dye ribbon that forms be deformed by the currents in the tank? And what if you added twice as many ice cubes, would the currents be twice as fast?
This is pretty much the easiest experiment you can imagine. If you are afraid of what food dye might do in the hands of your participants, you don’t even need to let them handle it themselves, even when they are working in small groups with individual tanks: just go around dripping the dye in and then add the dyed ice cubes yourself. While someone might still tip over a tank and spill the water, this has yet to happen to me. Especially since, before running the experiment, you will have pointed out that they need to make sure not to bang against the tables as to not disturb the experiment. And now apart from making sure that the ice cubes are frozen when you want to run this experiment, there is nothing that can go wrong. So why not try this experiment next time you want to talk about global ocean circulation?
Watch a video of the experiment here:
What would I do differently next time?
Next time, I would pay attention to which end of my tank will represent the equatorward and poleward side of the ocean. Not that it matters much, but in most graphics of sections through the North Atlantic, the northern end will be on the right side and the southern end on the left. If the experiment is set up the other way round (as on all pictures and movies above) you will need to remember to point it out (or even mark it on the tank with a sharpie or such).
Still scared of the hassle of running experiments?
And for all of you who hesitate doing awesome experiments because it looks like you need so much equipment: No you don’t. Here is a “making of” shot from how I did this experiment on my coffee table while sitting on my couch. The background is the back of an old calendar sheet, clipped to the back of a chair. And that’s it.
—
P.S.: This text originally appeared on my website as a page. Due to upcoming restructuring of this website, I am reposting it as a blog post. This is the original version last modified on November 4th, 2015.
Temperature-driven overturning experiment – the easy way
In my last post, I showed you the legendary overturning experiment. And guess what occurred to me? That there is an even easier way to show the same thing. No gel pads! (BUT! And that is a BIG BUT! Melting of ice cubes in lukewarm water is NOT the process that drives the “real” overturning! For a slightly longer version of this post check this out).
So. Tank full of luke warm water. Red dye on one end. Spoiler alert: This is going to be the “warm” end.
Now. Ice cubes on the “cold” end. For convenience, they have been dyed blue so that the cold melt water is easily identifiable as cold.
A very easy way to get a nice stratification! And as you see in the video below, awesome internal waves on the interface, too.
And because I know you like a “behind the scenes”:
I took this picture sitting on my sofa. The experiment is set up on the tea table. The white background is a “Janosch” calendar from 15 years ago, clipped to the back of a chair. And that is how it is done! :-)